Application of the INFOGEST Protocol Using the BioXplorer 100 for Automated Digestion Simulation
Sarah Verkempinck1, Tara Grauwet1,Dr. Mario Toubes-Rodrigo2
1Laboratory of Food Technology, Department of Microbial and Molecular Systems (M2S), KU Leuven, Kasteelpark Arenberg 22, PB 2457, 3001 Leuven, Belgium
2H.E.L Group, Unit 2 Centro, Boundary Way, Hemel Hempstead, HP2 7SU, UK
Table of Contents
Abstract
Digestion involves the breakdown of food into smaller compounds to facilitate nutrient release and absorption along the digestive tract. Studies have highlighted the significant impact of digestion on health, which led to the development of a variety of in vitro digestion protocols that produced non-comparable and often inconsistent results. In response, the international INFOGEST network was established in 2011 under a European Cooperation in Science and Technology (COST) Action [FA1005] to harmonize these protocols, enhancing the comparability and reproducibility of in vitro digestion studies. This Application Note presents a standardized approach for the application of the static INFOGEST protocol using the capabilities of BioXplorer 100. Our findings revealed no significant differences in terms of protein and lipid digestion from an Ensure® Plus Vanilla when using the INFOGEST protocol in test tubes and the BioXplorer 100. This confirms that the automated system can replicate the outcomes of digestion simulations in test tubes. These results highlight the capability of BioXplorer 100 to reduce human error through automation. The continuous monitoring and correction of critical parameters like temperature and pH ensure robust and reproducible data, supporting INFOGEST’s goal of standardized digestion simulations. Overall, the BioXplorer 100 offers a reliable, efficient alternative to manual digestion methods, advancing the field’s ability to produce consistent and accurate results across studies. This advancement promotes broader applications in in vitro digestion research and enhances our understanding of digestive processes.
Introduction
Digestion is a complex process that involves the breakdown of food from large polymers, including protein, lipids, and polysaccharides, into smaller compounds1. The aim of digestion is to release nutrients from the food matrix which in turn can be absorbed along the digestive tract. To achieve this goal, the process spans several phases, starting with mastication and mixing with saliva in the mouth, to highly acidic conditions in the stomach, to the nutrient, salt, and water absorption in the small and large intestine2.
In recent years, there has been a growing interest in understanding the digestive process. Studies have shown that health and disease are impacted by the way food is digested3. However, results regarding digestion simulation can be affected by the lack of homogeneity and standardization in protocols4. As a response, INFOGEST was founded in 2011 as a European Cooperation in Science and Technology (COST) Action aiming to harmonize in vitro protocols used by the scientific community working on digestion simulation, improving the comparability and reproducibility of results across studies. This collaborative effort has led to the development of widely accepted in vitro protocols, both for static and semi-dynamic approaches. In static models, physiological parameters, such as pH and enzyme concentration, are set and maintained during the experiment9. This approach is simple and cost-effective.
In this application note, we show how H.E.L’s BioXplorer 100 can be used to apply the static INFOGEST protocol.
Material and methods
The BioXplorer 100 is a multireactor system with 8 zones that can be controlled individually, including temperature and agitation. Additionally, pumps are connected to each reactor and can supply fluids. Each individual reactor was equipped with a pH and temperature process, sampling port, and 4 feeding lines. These feeding lines supplied simulated gastric fluids (SGF), NaOH, enzymatic mix, or simulated intestinal fluids (SIF). The reactors were agitated with marine propellors. The system is controlled by H.E.L’s WinISO.
Solutions and enzymes
The digestion of protein and lipids was tested using a convenient, liquid food Ensure® Plus Vanilla acquired from Sorgente (The Netherlands) and stored at room temperature until used, following the shelf-life recommendations. The composition of this drink was 4.92% lipids, 20.20% carbohydrates, 6.25% protein according to its label9.
Table 1 shows the concentration of the different (stock) solutions to be used during in vitro simulations according to the Brodkorb et al.2 protocol.
Table 1. Electrolyte solutions used in the digestion simulation. eSSF – Simulated Saliva Fluid, eSGF – Simulated Gastric Fluid, eSIF – Simulated Intestinal Fluid. Taken from Brodkorb et al2.
Porcine pepsin (3344 U mg-1), pancreas pancreatin (4xUSP) (amylase 41 U mg-1, lipase 36.3 U mg-1), trypsin (3.2 U mg-1), chymotrypsin (50.6 U mg-1) were acquired from Sigma Aldrich (Belgium).
Static conditions simulated
Table 2 shows the physical parameters and the addition of different solutions to the simulation process. Step 1 covered the oral dilution and equilibration phase, which was performed for 10 minutes at 35°C and 250 rpm. No digestive enzymes were added in this step, as mainly salivary amylase is of importance during oral simulation, yet limited starch was present in the Ensure drink. The rest of the steps were performed at 37°C and 250 rpm. In step 2 (gastric phase fluid addition), the total SGF volume and pepsin volume were pumped into the vessel at maximum speed before proceeding to step three. SGF master mix was prepared according to the suggestions of Brodkorb et al. (2019) and included eSGF (pH 3), 0.3M CaCl2, 2M HCl, and milliQ water. The ratio of HCl to milliQ water added to the SGF mix was determined in a preliminary experiment to reach a pH of 3 of the digest during the simulation of the gastric phase. No gastric lipase was added at this stage since the majority of lipids are digested in the intestinal phase. In step 3 (gastric phase simulation), no solutions were added, but maintained temperature and agitation to allow substrate-enzyme interaction for maximally 2 hours. In step 4 SIF solution was added. SIF contained eSIF (pH 7), 0.3M CaCl2, and bile salts (10 mM final concentration) prepared in MilliQ water. In step 5, NaOH (2 M) was pumped to increase the pH to 6.6 which gradually increased further in the subsequent steps until a pH value of 7 was reached during the simulation of the small intestine. In step 6, the pancreatic enzyme solution was added using a cooled syringe pump and contained 100 U ml—1 trypsin, 25 U ml—1 chymotrypsin, 200 U ml—1 amylase, and 177 U ml—1 lipase. Indeed, one of the pumps was equipped with a cooling mantle to keep the enzymatic solution at 4°C to preserve the enzyme activity throughout digestion simulation. Finally, in step 7, the simulation of the small intestinal phase was performed during which no solutions were added, but temperature and agitation were maintained to allow substrate-enzyme interactions for maximally 2 hours.
Digestion was simulated in the available eight individual reactors. This allowed to perform a kinetic digestion approach, meaning that the time-dependent digestion behavior could be evaluated from independent recipients. Based on insights in previous work, the following time points were selected to be evaluated in terms of digestion product formation: 5; 10; 20; 30; 45; 60; 90; and 120 min after enzyme addition in the gastric or small intestinal phase. When gastric phase kinetics were studied, the pepsin activity was stopped by increasing the pH to 8 at the pre-determined moments. In the small intestinal phase, an aliquot of the digest was inhibited by adding 4-bromophenylboronic (1 M in methanol) to inhibit pancreatic lipase while another aliquot was placed in a water bath (5 min, 98 °C) to inhibit proteases and amylase. After enzyme inhibition, the digest (aliquots) were placed in an ice bath.
The protein and lipid content throughout the simulation was quantified following the methodology
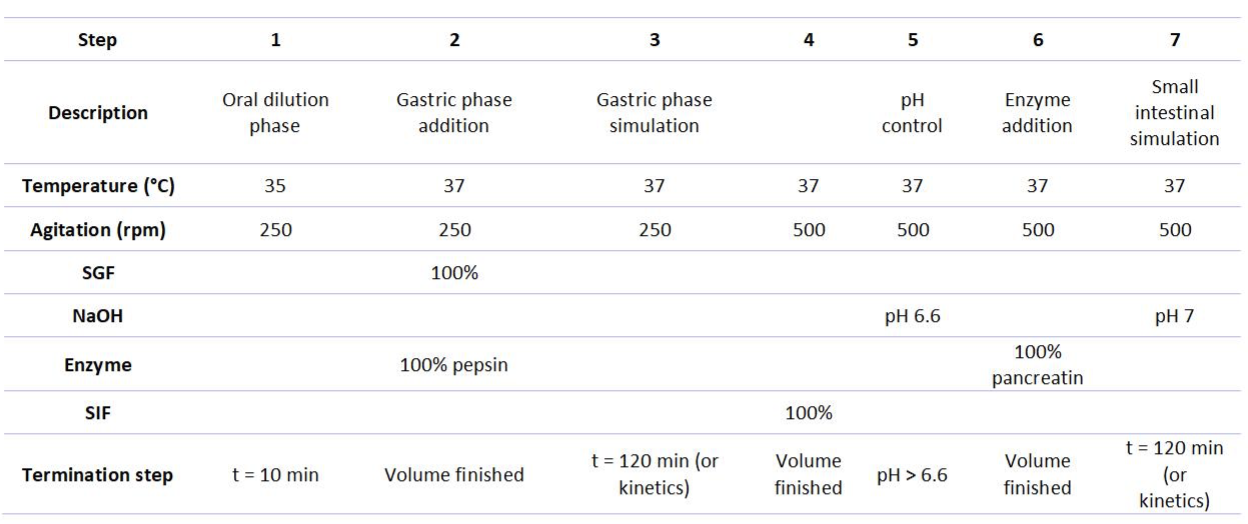
Table 2. Physicochemical parameters and solutions added in the different steps of the digestion simulation
Results and Discussion
The evolution of accessibility of protein in Ensure® Plus Vanilla was evaluated using two approaches: a manual method with 10 ml tubes as described in the INFOGEST protocol2, and an automated method using the BioXplorer 1009. The manual tube method allowed testing of a total volume of 10 ml, whereas the BioXplorer 100 handled 80 ml. A key difference between these approaches lies in agitation and temperature control. In the tube method, samples were agitated on rotating wheels in a 37°C incubator. In contrast, the BioXplorer maintained a stable temperature of 35°C during the equilibration step and 37°C during effective digestion simulation (remaining steps) , with constant agitation at 250 rpm during the gastric phase and 500 rpm during the small intestinal phase.
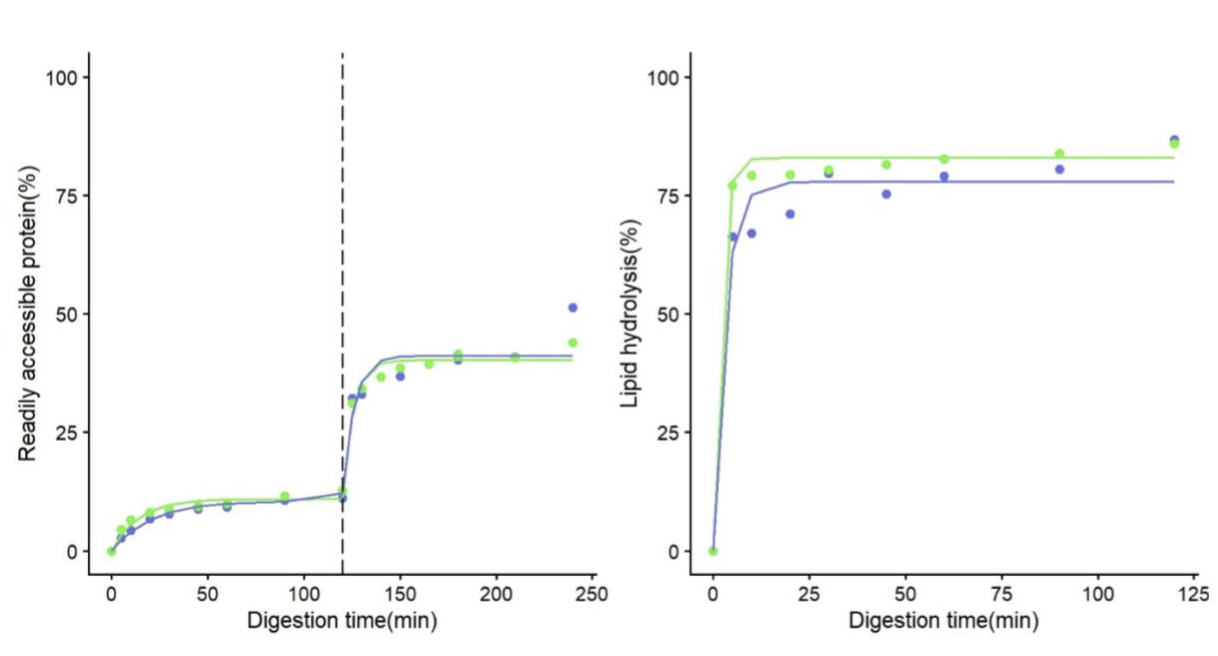
Figure 1. Evolution of the readily accessible protein and lipid in the simulation during the Gastric Phase (before 120 min) and Small Intestine phase (after 120 min). The dashed line represents the shift between phases. Colors represent the mean of testing (blue for tube, green for BioXplorer). Circles represent measured (circles) versus predicted values (solid line)
Figure 1 illustrates the evolution of readily accessible protein and protein hydrolysis during the simulated process in tubes and BioXplorer. Around 12% of the protein was made available by the end of the gastric phase, reaching up 44-51% at the end of the small intestinal phase. Lipolysis was much faster, reaching values over 60% after 5 mins of pancreatic lipase addition. No apparent differences were observed between the tube and BioXplorer results. This indicates that the INFOGEST protocol can be effectively implemented in the BioXplorer 100. The automated process in the BioXplorer minimizes human handling, reducing potential errors .The ability to continuously monitor and correct critical parameters such as temperature and pH ensures robust and reproducible data, aligning with the goals of the INFOGEST community effort to harmonize digestion simulations.
Conclusion
The results shown in this Application Note underscore the efficacy of the BioXplorer 100 in implementing the INFOGEST protocol. The capabilities of this system enable automated control implementation, providing a reliable and efficient alternative to manual methods.
References
1. Grundy, M. M., Moughan, P. J. & Wilde, P. J. Bioaccessibility and associated concepts: Need for a consensus. Trends Food Sci. Technol. 104373 (2024).
2. Brodkorb, A. et al. INFOGEST static in vitro simulation of gastrointestinal food digestion. Nat. Protoc. 14, 991–1014 (2019).
3. Ross, F. C. et al. The interplay between diet and the gut microbiome: implications for health and disease. Nat. Rev. Microbiol. 1–16 (2024).
4. Colombo, R., Ferron, L., Frosi, I. & Papetti, A. Advances in static in vitro digestion models after the COST action Infogest consensus protocol. Food Funct. 12, 7619–7636 (2021).
5. Zhou, H., Tan, Y. & McClements, D. J. Applications of the INFOGEST in vitro digestion model to foods: A review. Annu. Rev. Food Sci. Technol. 14, 135–156 (2023).
6. Ménard, O. et al. Static in vitro digestion model adapted to the general older adult population: an INFOGEST international consensus. Food Funct. 14, 4569–4582 (2023).
7. Mulet-Cabero, A.-I. et al. A standardised semi-dynamic in vitro digestion method suitable for food–an international consensus. Food Funct. 11, 1702–1720 (2020).
8. Minekus, M. et al. A standardised static in vitro digestion method suitable for food–an international consensus. Food Funct. 5, 1113–1124 (2014).
9. Verkempinck, S. et al. Studying semi-dynamic digestion kinetics of food: Establishing a computer-controlled multireactor approach. Food Res. Int. 156, 111301 (2022).