Mitigate Thermal Runaways
How can we mitigate the risks of thermal runaways?
Thermal runaways, once initiated, can lead to the rapid release of stored energy in the form of heat, potentially resulting in a fire or an explosion. Although prevention of such a process should always be a priority, under certain conditions, thermal runaways will occur, and it is imperative to mitigate the risks associated with it.
In previous sections we have extensively discussed how we can understand the process: understanding the conditions that will result in thermal runaways, such as the chemical components (anode and electrolyte), possibility of internal short-circuits (for example, dendritic growth at low temperatures), and external factors (such a high temperature, or mechanical damage).
Adiabatic calorimeters can help to understand what the consequences of thermal runaways are. Adiabatic calorimetry can help to determine what the temperature at which the thermal runaways might occur (exotherm) and the temperature that the battery can reach. Propagation tests allow us to characterize how the thermal runaway can affect the neighboring cells and understand the risks of cascading failures.
However, assuming that the worst could happen can help to design methods to mitigate the potential damage that thermal runaways can inflict.
Mitigation strategies
Thermal Management Systems: this strategy is based on removing heat, avoiding reaching high temperatures in hot spots that initiate the thermal runaway. In order to achieve temperature uniformity, strategies such as active systems (e.g., liquid or air cooling) and passive (phase changing material, heat spreaders) may be used.
Cell Design and Separators: as mentioned in previous sections, separators act as physical barriers between the electrodes. These structures activate at high temperatures, shutting down the ionic flow and stopping the battery from functioning. One of the strategies used in the separator is using structures that physically change under thermal runaway risk, closing down the pores.
Battery Management Systems (BMS). A BMS monitors and manages the battery’s operation, ensuring it remains within the limits of the Safe Zone. If dangerous conditions are met, BMS can disconnect the battery from the load or source.
Physical Barriers: in multi-cell batteries, physical barriers or fire-retardant materials can be placed between cells to prevent or slow thermal propagation.
Solutions
The iso-BTC and iso-BTC + allow for thermal mapping during testing, highlighting the regions of the battery that are more susceptible to generating heat. This information can be used to design and implement efficient thermal management strategies. Additionally, using the information supplied by BTC-130 and BTC-500 can help predict the battery’s thermal behavior. Detecting decomposition temperatures and the resultant heat released can help understand the thermal runaway from the process. BTC-500 can be further used to initiate physically-induced thermal runaways and capture the event unfolding using its integrated camera.
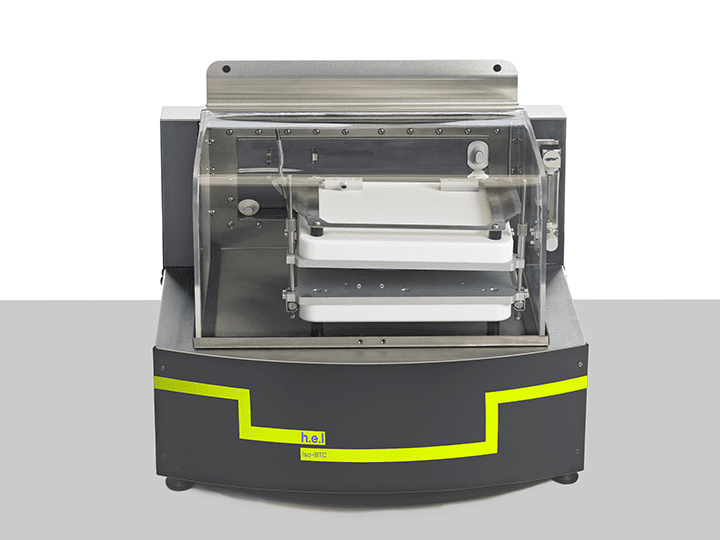
iso-BTC | Bench-top, battery performance testing, isothermal calorimeter
The iso-BTC (Battery Testing Calorimeter) is an isothermal calorimeter designed for the ch...
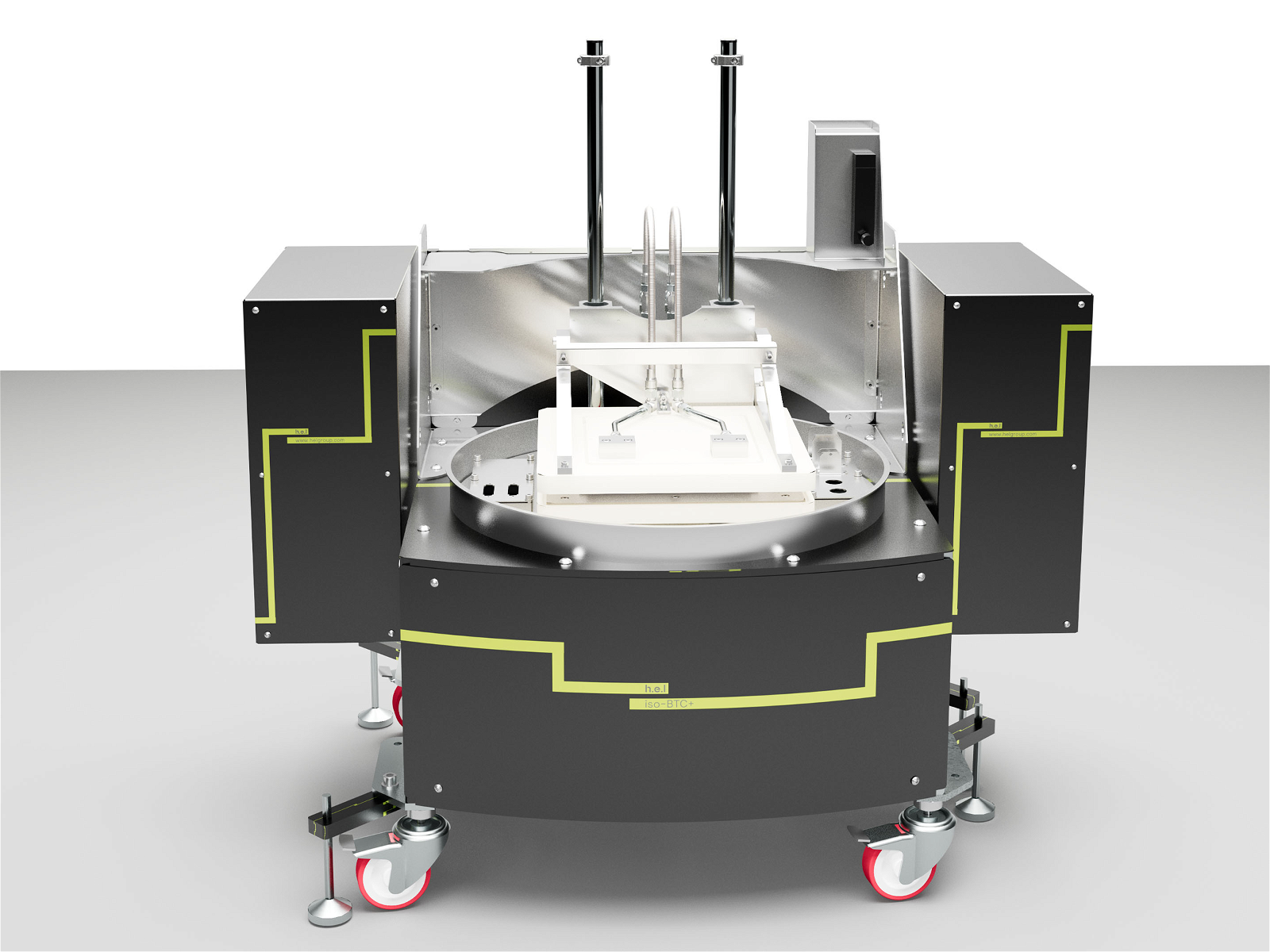
iso-BTC+ | Floor standing, battery performance testing, isothermal calorimeter
Faster product development, testing, and launch through scientifically robust data: R...
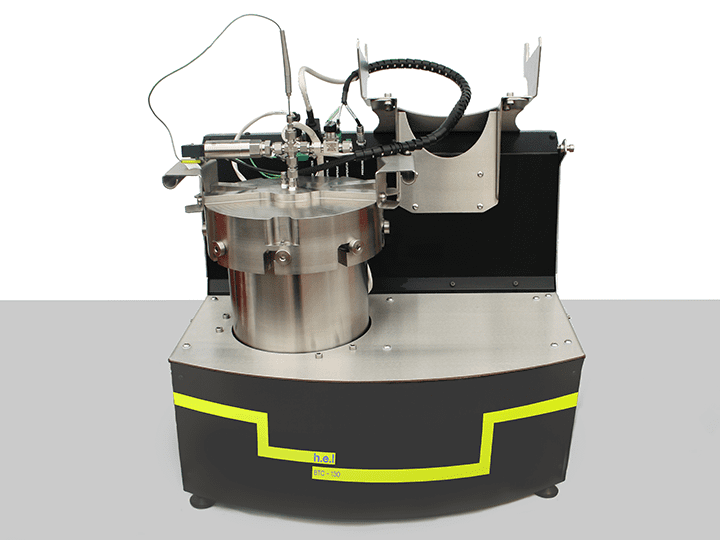
BTC-130 | Bench-Top, Battery Safety Testing, Adiabatic Calorimeter
The BTC-130 (Battery Testing Calorimeter) is a bench-scale adiabatic calorimeter designed ...
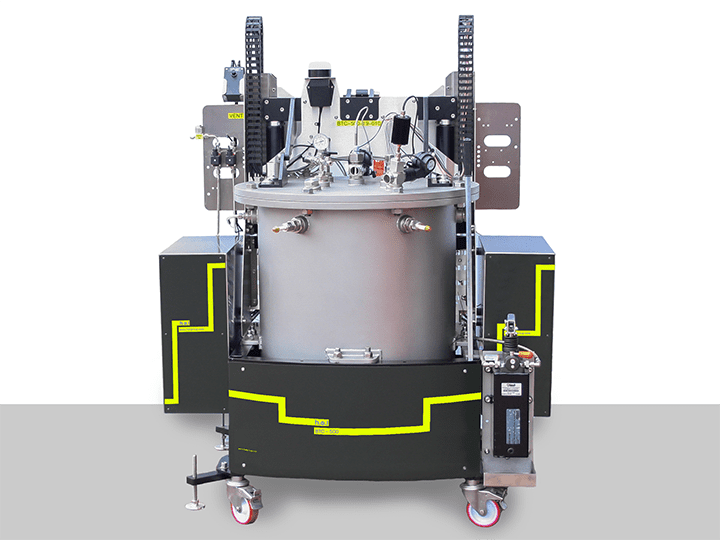
BTC-500 | Large-scale, battery testing, adiabatic calorimeter
The BTC-500 (Battery Testing Calorimeter) is a floor-standing adiabatic calorimeter for th...