Achieving safe conditions for hydrogenation reaction using concentrated raw materials
Matteo Pori1, Mario Toubes-Rodrigo2
1 BASF, Via Pila, 6/3, 40037 Pontecchio Marconi BO, Italy
2 H.E.L Group, Unit 2 Centro, Boundary Way, Hemel Hempstead, HP2 7SU
Table of Contents
Abstract
Hydrogenation involves the addition of hydrogen gases into molecules, transforming bonds (e.g. C=C to C-C). Hydrogen is highly flammable and explosive, and as such, hydrogenation reactions and their products need to be tested in advance. In this application note, we present the thermal behavior of an alkane and alkene involved in the hydrogenation reaction measured in a Phi-TEC I. Results showed that the alkene was susceptible to decomposition reactions when it reached a temperature 238.8 °C, where the alkane did not display such behavior. Phi-TEC I is an adiabatic calorimeter that has shown its capabilities to detect thermal events in reactants involved in risky hydrogenation reactions.
Introduction
Hydrogenation is the chemical reaction that involves the addition of hydrogen gas (H2) to an unsaturated moiety. This reaction results in the addition of one or more hydrogen atoms into the molecule structure, transforming C=C bonds into C-C1. Other functional groups are susceptible to catalytic hydrogenation, such as C=O, and NO2,2 As such, the product of the hydrogenation is the formation of alkanes from alkenes. However, for this reaction to occur, catalysts are necessary. Heterogenous catalysts are the predominant type of catalyst used for this type of reaction, and palladium is the dominant catalyst metal 1. One of the main safety concerns for any hydrogenation reaction is fire and explosions due to the pyrophoric nature of the catalysis, the potential instabilities of reagents and products, the use of flammable solvents and hydrogen, and the high-pressure conditions 2. As such, it is fundamental to understand the properties of hydrogen, catalysts, and reagents and products2. This situation is worsened when using over-pressuring conditions, and as such, it is critical to perform in-depth risk assessments. In this application note, two chemicals were analyzed in order to characterize the risk associated with their decomposition at high temperatures using adiabatic calorimetry. The objective of this characterization is to increase the concentration of the reagents from 48% (current conditions) to 60% to increase the output of the process. As such, a 60% alkane and 60% alkene solution were adiabatically tested.
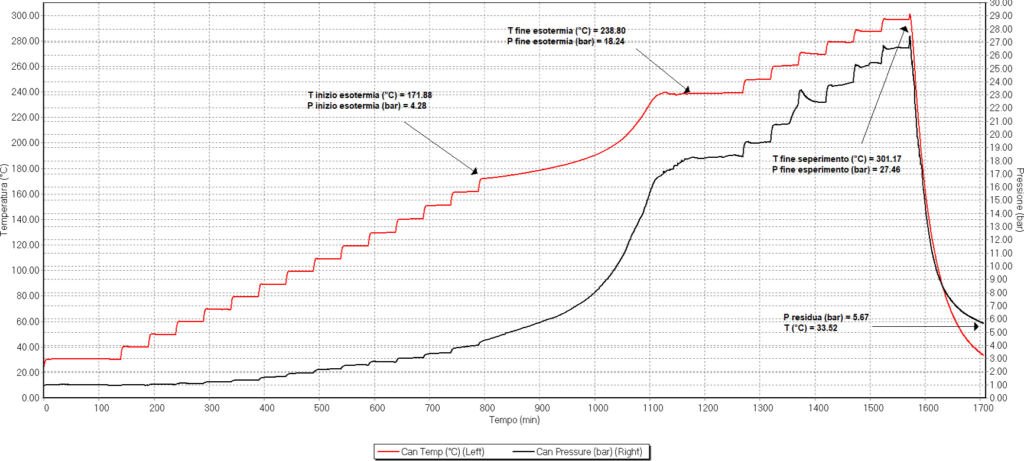
Figure 1. Phi-TEC I analysis of 60% alkene solution
Preliminary Data
As mentioned in the introduction, the previous conditions for the process involved the use of reagents at 48% had been characterized. The energy released by the reaction was measured by heat flow calorimetry with a total value of -184.1 kJ·kg-1. The theoretical value for reaction enthalpy at 60% increased to -237.2 kJ·kg-1, and the maximum temperature of synthesis reaction (MTSR) of 205°C.
Sample | Cp at 30°C | Cp at 90°C | Average |
---|---|---|---|
kJ/(kg·K) | kJ/(kg·K) | ||
Alkene at 60% | 2.01 | 2.08 | 2.04 |
Alkane at 60% | 1.99 | 2.18 | 2.08 |
Table 1. Specific heat measured twice and averaged calculated by reaction calorimetry of alkene and alkane solutions at 30°C and 90°C
Characterization of the heat capacity of the 60% solution was calculated by reaction calorimetry. This was measured at two relevant temperatures: 30°C and 90°C, and the average was calculated. (Table 1)
Material and methods
Two solutions were evaluated: one containing 60% of alkane and 60% of alkene. The decomposition reaction was evaluated using a Phi-TEC I equipped with 4A high-pressure stainless steel 316 test cells. The total volume of the cell was 10 ml, with a Phi-factor of 1.96-1.97. The Phi-TEC I was operated in heat-wait-search (HWS) mode. The testing cells were filled to over 80% of their capacity with the chemicals of interest (Table 2).
The starting temperature was 30°C with a maximum search temperature of 300°C and maximum track temperature of 400°C. Search temperature was increased by 10°C with a searching time of 10 mins. The maximum investigation temperature was set at 120 bar.
The self-heating sensitivity of the system is 0.03°C/min. The experiments was performed under stirring.
Sample | Sample Weight (g) | Degree of Filling (%) |
---|---|---|
Alkene | 7,36 | 81,8 |
Alkane | 7,28 | 80,9 |
Table 2. Characteristics of the solutions added to the test cells.
Results and Discussion
Alkene at 60%
The exotherm was detected starting at 171.9°C and a pressure of 4.3 bars. The exotherm progressed until it reached a temperature of 238.8°C and 18.2 bar of pressure. The adiabatic temperature rise (ATR) corrected accounting for the thermal inertia was 131.8°C, and a corrected final temperature of 303.7°C. The reaction enthalpy corresponding to the decomposition reaction was 269.6°C. The residual pressure at 25°C was 5.3 bar, and the pressure increase at 25°C was 4.3 bar. The amount of incondensable gas produced during the reaction was 3.2·10-4 mol.
Alkane at 60%
Differently to concentrated alkenes, in the case of the alkane, not a single exotherm was detected. Instead 4 of them were identified. The first one started at 167.9 °C at 4.6 bar, concluding at 173.2 °C and final pressure of 4.9 bar. This corresponded to an adiabatic temperature rise of 10.3°C and a corrected final temperature of 178.2°C. The reaction enthalpy of this first reaction was 21.5 kJ/kg. A second exotherm was detected starting at 182.6°C and 5.2 bar, reaching final values of 188.4°C and 5.7 bar. The corresponding values for the ATR and corrected final temperature were 11.3°C and 193.9°C, with a reaction enthalpy of 23.6 kJ/kg. The third thermal events occurred at 188.7°C and 5.8 bar of pressure, reaching final values of 195.2°C and 6.3 bar. The ATR corresponding to this exotherm was 12.6°C and a the final corrected temperature was 201.4°C, with a reaction enthalpy of 26.3 kJ/kg. The fourth and final exotherm was detected at a temperature of 205.8°C and 7.3 bar, reaching final values of 210°C and 8.4 bar. The corresponding ATR was 8.2°C and the corrected final temperature reached a value of 214.1°C. The enthalpy value was 17.2 kJ/kg.
Comparison between the thermal decomposition of alkane and alkene
When comparing the decomposition of alkane and alkene, the difference was clear. When the alkane was exposed to increasing temperatures, a clear exotherm was detected starting at 171.9°C increasing to a final temperature of 238.8°C, with a corrected value of 131.8°C. On the other hand, the alkene exotherm started at a 167.9°C. However, this exotherm was so small that Phi-TEC I interpreted it as completed. As a result, 4 different very small concatenated exotherms were detected, reflecting a very slow and gentle slope.
Figure 2. Phi-TEC I analysis of 60% alkane solution. Temperature (red line) and pressure (black line) were measured.
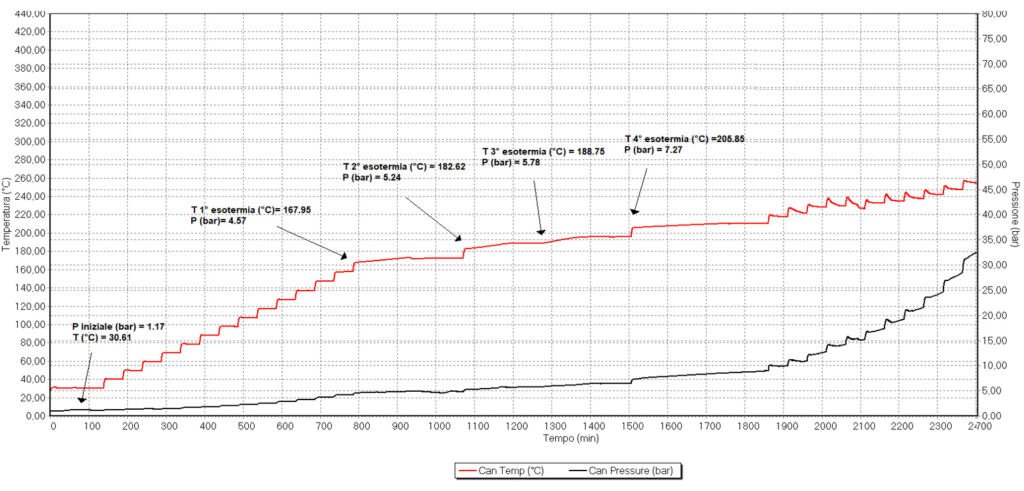
Figure 2. Phi-TEC I analysis of 60% alkane solution.
Conclusion
Adiabatic calorimetry experiments were conducted to assess the risk associated with the decomposition of two reactants involved in a hydrogenation reaction: one alkane, and one alkene. These two chemicals would be exposed at high temperature, so understanding their potential side reactions is fundamental to ensure safe conditions. The preliminary data revealed an increase in the reaction enthalpy with increased concentration of reactants. Results from adiabatic calorimetry demonstrated distinct thermal behaviors between alkene and alkane decomposition. Alkane decomposition exhibited clear exothermic behavior, while alkene decomposition showed small, concatenated exotherms, almost out of detection range. This comparison underscores the necessity of comprehensive risks assessments and safety protocols, especially when dealing with concentrated chemicals. Understanding the thermal behaviour of the reaction mixtures is crucial for mitigating potential hazards, such as fire and explosions in hydrogenation processes. Moving forward, these findings provide valuable guidance for optimizing reaction conditions to achieve higher outputs while maintaining safety standards. By leveraging adiabatic calorimetry and other analytical techniques, researchers and engineers can make informed decisions to ensure the safe and efficient operation of hydrogenation reactions using concentrated raw materials.
Bibliography
- Stoffels, M. A., Klauck, F. J., Hamadi, T., Glorius, F. & Leker, J. Technology trends of catalysts in hydrogenation reactions: a patent landscape analysis. Adv. Synth. Catal. 362, 1258–1274 (2020).
- Chandra, T. & Zebrowski, J. P. Hazards associated with laboratory scale hydrogenations. J. Chem. Health Saf. 23, 16–25 (2016).