The results of successfully functionalizing C-H bonds (CHB) are one of synthetic chemistry’s most important topics today. So says Prof. Debabrata Maiti on the process that transforms simple Carbon and Hydrogen into complex molecules in just a few stages. These qualities of CHB are catching the attention of some of today’s high-value sectors.
Let’s kick off with a handy definition. Functionalizing a CHB converts it to a C-R bond. Here, R represents a functional group that enables a final molecule to perform a specific reaction. So, any organic molecule, for example, the aromatic hydrocarbon Toluene or the nonpolar molecule Hexane, has many CHBs. The job of CHB functionalization is to try to establish how to selectively isolate and convert one CHB into a different type of bond. Do that, and you essentially transform a very simple molecule into something valuable.
These ‘molecules of interest’ can be life-saving drugs, agrochemicals, pharmaceuticals, or perfume molecules. Not only is their need widespread, but the raw materials required for the conversions are also the simplest and most readily available. This relatively low cost to high benefit ratio is an attractive feature that the industry hasn’t overlooked.
Taking on the dragon
Prof. Maiti is based at the Indian Institute of Technology (IIT) Bombay. The main challenge of his work is to seek something special that can convert these simple organic molecules into the complex. Cell selectivity is what we’re talking about here. This is a quest to find ‘the one’, that special carbon in the bond of multiple C-H sites, and use it to selectively promote a reaction at one center without touching another. That’s the quest, and the dragon you’re battling in this campaign is differentiation: what’s to separate these competing centers when they are all equally accessible and mirror the simplicity or complexity of all other centers around them?
It’s best to imagine this dragon having many heads, each wearing a crown of a different carbon-hydrogen bond. You want to look at each head individually without drawing attention from the others. Plus, you’ve got to keep yourself and the beast alive while this happens. In the lab is how you’re looking to find a living CHB that responds to the right catalysts and methods, one that avoids the problems of incorrect selection, which can result in scattergun reactions breaking out in various quantities.
Enzyme engineering carried out this way is more prevalent today than ever, but that doesn’t mean it’s a simple task. Prof. Maiti’s own CHB functionalization endeavors are aligned with the efforts of a worldwide scientific community. All are working to identify an efficient, lab-based selective bio-inspired catalysis.
Annoyingly and inspirationally, it’s a process that the natural world already does well. In natural biology, you can take one organic molecule and produce one identifiable result with it. The enzymes, typically protein molecules, are the organic catalysts here.
Attempt the same conversion in lab-based synthetic chemistry, and the tables turn. You may successfully replicate the reaction, but the result will most likely miss the required selectivity. This is especially the case when turning typically large and complex catalytic protein molecules into simpler and more efficient chemicals is harder.
Reigning in those substrates
Bio-inspired chemical enzyme reactions typically occur through a simple active site or metal center. Evolution over millions of years has provided enzymes with selectivity features, which means that enzymes will catalyze specific reactions using specific molecules, as these chemical substances will fit in the active center of the enzyme like a key in a lock. The immobilization of the substrate inside the enzyme and the proximity with the active center reduces that activation energy of the chemical reaction of interest, making, for example, Hydrogen bonding interactions possible, by electrostatic forces, CH−π, or one of many other weak interactions. In nature, you can expect to see hundreds or thousands of weak interactions with no movement impact. They are, instead, the perfect fit.
Lab-applied substrates are far less obliging. Instead of having physically constraining centers, which guides the molecule in a configuration that will make it susceptible to the chemical reaction, the chemical environment can tolerate degrees of freedom away from the active site. This allows them to rotate, travel upside down, and go any way they want. Prof. Maiti is working to counter this fluidity with a design that will enable them to discover the substrate’s orientation and whether it is fixed or permanently locked. Knowing that will make it possible for them to change the active site or metal center and consequently increase the yield and specificity of the reaction.
Diversifying the approach
The activation of C-H bonds requires the supply of energy. Traditionally, in industry, to achieve the activation energy needed to start the chemical reaction, high temperatures are regularly supplied – reaching values as high as 120, 150oC. This situation can be improved using the type of catalysts that Prof. Maiti is developing.
Another crucial step in the catalysis process is the catalyst regeneration, in this case, the metal center, which needs re-oxidizing (electrons need to be supplied to the metal)—the use of strong oxidizing chemicals, such as silver nitrate and silver acetate. However, from an industrial perspective, using these substances is not viable since they are costly and dangerous. Instead of using oxidizing salts, alternatives, for example, electrical currents (electrochemistry) or light sources (photochemistry), can be provided. Photocatalytic and electrochemical approaches are not perfect, but they make it possible to take a more targeted approach. These alternative processes have drawbacks since the incorrect light wave can be supplied, or the levels of electrical charges applied can be inappropriate. The process needs to oxidize the metal center to return it to the catalytically competent intermediate. A mixed alternative can be used, in which photocatalysts containing expensive metals, such as palladium, react with the light supplied and transfer electrons to the metal centers, regenerating the catalysts.
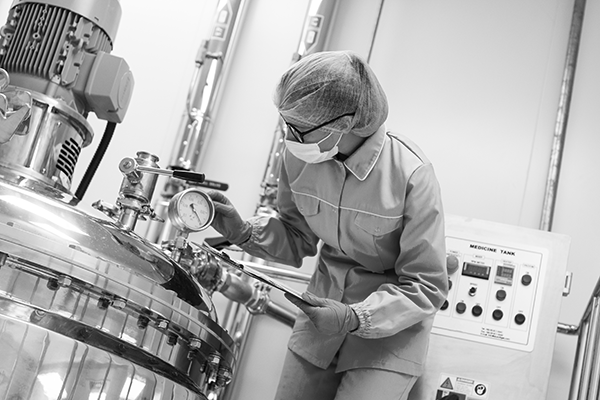
Worldwide interest
Various ways to achieve impactful CHB functionalization are currently being explored globally. Like the enzymes themselves, Prof. Maiti is aware that no methods are likely to be perfect. However, as a synthetic chemist, he hopes one day to discover a process that’s as effective as nature itself. High hopes perhaps, but for him, efficiently produced CHB conversions achieved at an industrial scale would prove revolutionary.
And it seems that the positive effects of these conversions are beginning to be felt. Already pharma companies can reduce timescales and synthesis stages, essentially changing the way molecules are prepared. Some multi-step processes are now impressively reduced, as ten or twenty stages are cut down to a handful.
And the benefits are showing themselves to be relevant across sectors too, where successful molecule findings where inter-industry sharing is taking place. For example, developing a method to work on phenol or Toluene with a simple molecule that’s widely available, like benzoic acid, benefits both pharmaceutical and agrochemical interests.
So it seems that Prof. Maiti may have a point. We may not be there just yet, but there are plenty of reasons to be optimistic about the possibilities of CHB functionalization.